Gels are interesting materials from many perspectives. Their ability to absorb large amounts of solvent results in a range of attractive material behaviors. Hydrogels are characterized by the fact that they absorb water, in contrast with organogels, which gelate organic solvents. Hydrogels can further be classified as natural or synthetic, based on the gelator (high molecular weight, i.e. polymeric, or low molecular weight, i.e. small molecule), on their physical structure (i.e. amorphous, semicrystalline or crystalline) or on the nature of the network (permanently or physically crosslinked).
This versatile class of materials has a wide range of applications. Hydrogels are found in personal healthcare products such as toothpaste, hair gel, contact lenses for example. More advanced applications include wound dressing, pharmaceutical formulations and wastewater treatment. Due to their ability to form 3D networks with customizable properties such as tensile strength, mesh size and the possibility to include functional moieties, hydrogels also form an interesting medium for tissue engineering.
The material properties of hydrogels depend on various factors such as the chemical composition, the nature of the fibers, the network that is formed by the fibers and the degree of swelling. Understanding and further developing a hydrogel requires insight in all these factors, which span over multiple decades of length scales. Therefore, a versatile toolbox of analysis techniques is required. At the smallest level, chemical composition and assembly on the molecular level can be addressed with techniques such as UV-vis spectroscopy and NMR (Nuclear Magnetic Resonance). In the medium size range, microscopy plays an important role in the visualization of the structures that form the network. Apart from fibers, these can be platelets, vesicles, tubuli or helixes. On the largest length scale, that of the network, rheology is an invaluable tool. [1]
SAXS and WAXS analysis of hydrogels
In addition, and complementary to the above-mentioned techniques, the analysis over multiple decades in length can be done simultaneously using Small and Wide-Angle X-ray Scattering (SWAXS). The smallest length scales are addressed with wide-angle scattering, which gives insight into the ratio between amorphous and crystalline material as well as details of the crystalline phase and unit cell packing [2]. Information about larger length scales is accessed through scattering at medium and small angles, which reveals fiber cross-section and flexibility and allows to extract parameters about the mesh size of the gel [3].
An elegant example of the use of WAXS and SAXS for the analysis of a hydrogel is the work of Giuri et al. in the frame of a collaboration between scientists from the university of Bologna, university of Glasgow and university of Bristol [4]. In this article, a supramolecular hydrogel is formed by the gelation of (2S)-2-(2S)-2-{2-naphthalen-2-yloxyacetamido}-3-phenylpropanamido-3-phenylpropanoic acid (2NapAA). Using SAXS and WAXS, they follow the transition from the solution to the gel phase, and the subsequent crystallization.
The gels are prepared by the addition of glucon-δ-lactone (GdL) to a stock solution 2NapAA. Glucon- δ-lactone slowly hydrolyses, the resulting acid induces the slow gelation of the functionalized dipeptide. Phase characterization was performed on gels with two levels of GdL concentration: 4 mg/ml or 8 mg/ml.
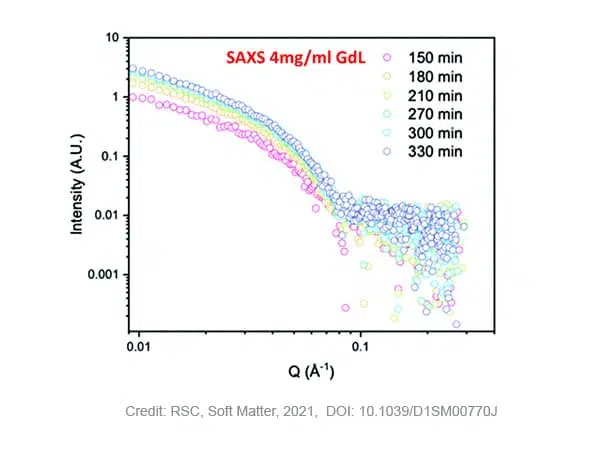
SAXS measurements on samples with 4 mg/ml GdL show that stable gelation occurred with an increase of the scattering intensity over time and maintained structured pattern. Fitting of the curves, results in a fibre radius of 2.9 nm in the beginning of the process, evolving to a maximum of 4 nm after 210 minutes. After this time, the radius does not further increase while the pH of the solution still decreases due to the slow hydrolysis of GdL. The Kuhn-length, a measure for the flexibility of the system, steadily increases over time from ~5 to ~20 nm, indicating that the fibres become more rigid. Moreover, no crystallisation of the self-assembled fibers was detected during the timescale of the SAXS experiment as no WAXS data peak was observed in that time.
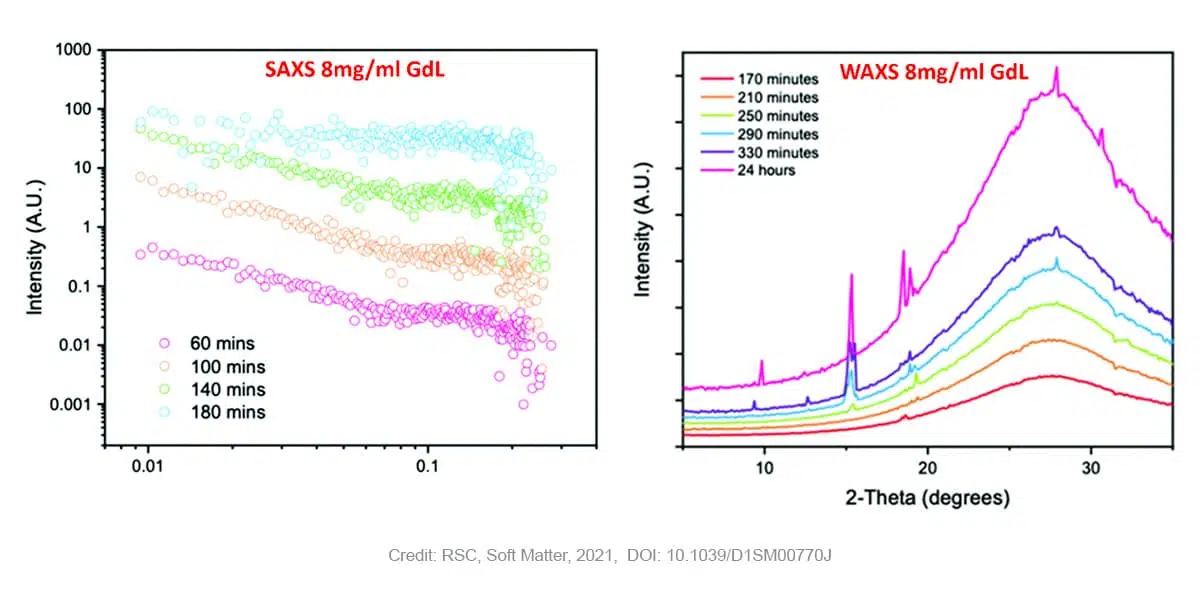
In case of gels prepared with higher concentrations of GdL (8 mg/ml) SAXS measurements still reveal the presence of fibers at early points, however in that case the Khun length is not detected anymore suggesting a much higher dimension and already stiffen fibers. Moreover, a subsequent loss of the SAXS signal is observed over time as the pH drops further. The WAXS data shows no peaks early on in the process, suggesting an absence of ordered molecular packing. After 170 minutes, the first peak starts to appear at a q value of 1.32 Å-1 corresponding to a real space value of 4.6 Å. This appearance of ordered structure in WAXS (i.e. at short length scales) coincides with the loss of structure in SAXS (i.e. at long length scales). This observation is indicative of the crystallization and consequent degradation of the gel network. Previous research [5] has shown that the distance of 4.6 Å corresponds with the 111 plane, indicating that crystal growth starts along the fibre axis. Over time, more peaks corresponding to the same crystal phase appear.
The combined SAXS and WAXS results unambiguously show that the gel phase and the crystal phase are two distinctly different phases. In the gel-phase (at early time points), the WAXS data shows that there is no molecular order. Moreover, when molecular order starts to appear, as evidenced by the WAXS patterns, the SAXS data indicates that there is no long-range order anymore.
In conclusion, the SWAXS data uniquely allows to study the packing in both the gel and the crystal phase. For the gel under investigation, the data shows that the packing is different for both phases. This is an important finding as many studies assume that the packing of the crystal phase is the same as the packing of the gel. This data clearly shows you cannot translate one packing to the other without caution.